Going with the flow of thermal regulation… Biomimicry at Thales, episode 1
What if we solve society's problems by looking at nature's toolbox? Time to zoom in on some of nature's best ideas, starting with the art of thermal regulation. How can we keep ourselves, our buildings and our equipment either warm or cool, in the most efficient manner possible?
Thales Hardware Architect Wessel Wits holds out small a 3D-printed object for us to inspect. The object is covered in the grooves and swirls that we normally associate with those in a human brain – or, in this case, of brain coral. The ridges and furrows are not for show; every twist and curve helps guide the flow of ocean water along the surface of the coral, in order to maximise the amount of coral surface touched.
Termite mound airflow
The briny sea water is filled with the coral’s natural nutrients, small organisms such as zooplankton, which its filter feeds through its exposed surface. The coral’s spheroid shape and grooved surface make it easier for water to flow into and then remain inside the run of the passageways, for maximum feeding opportunities. Wits explains that you can find these kinds of design all over nature. “Termite mounds have a similar design, where the bottom will contain passageways optimised to draw in cool air, which is then sent through a chimney-like system of channels, to allow easy airflow along the surfaces that efficiently cools down the entire structure by natural convection.”
Wits, working together with partners from the University of Twente and Optimal Thermal Solutions B.V., 3D printed several structures designed to mimic similar water and airflow circulation. They used selective laser-sintered polyamide heat sinks for experimental airflow testing, and selective laser-melted aluminium heat sinks for thermal performance testing. “It proved that nature’s design provides extremely efficient thermal regulation! The next step would be to optimise structures for forced convection. For instance, when pumping around cooling liquid or coolants to cool down a radar, or to find ways to withdraw heat from a surface in order to keep an entire structure at a uniform base temperature.”
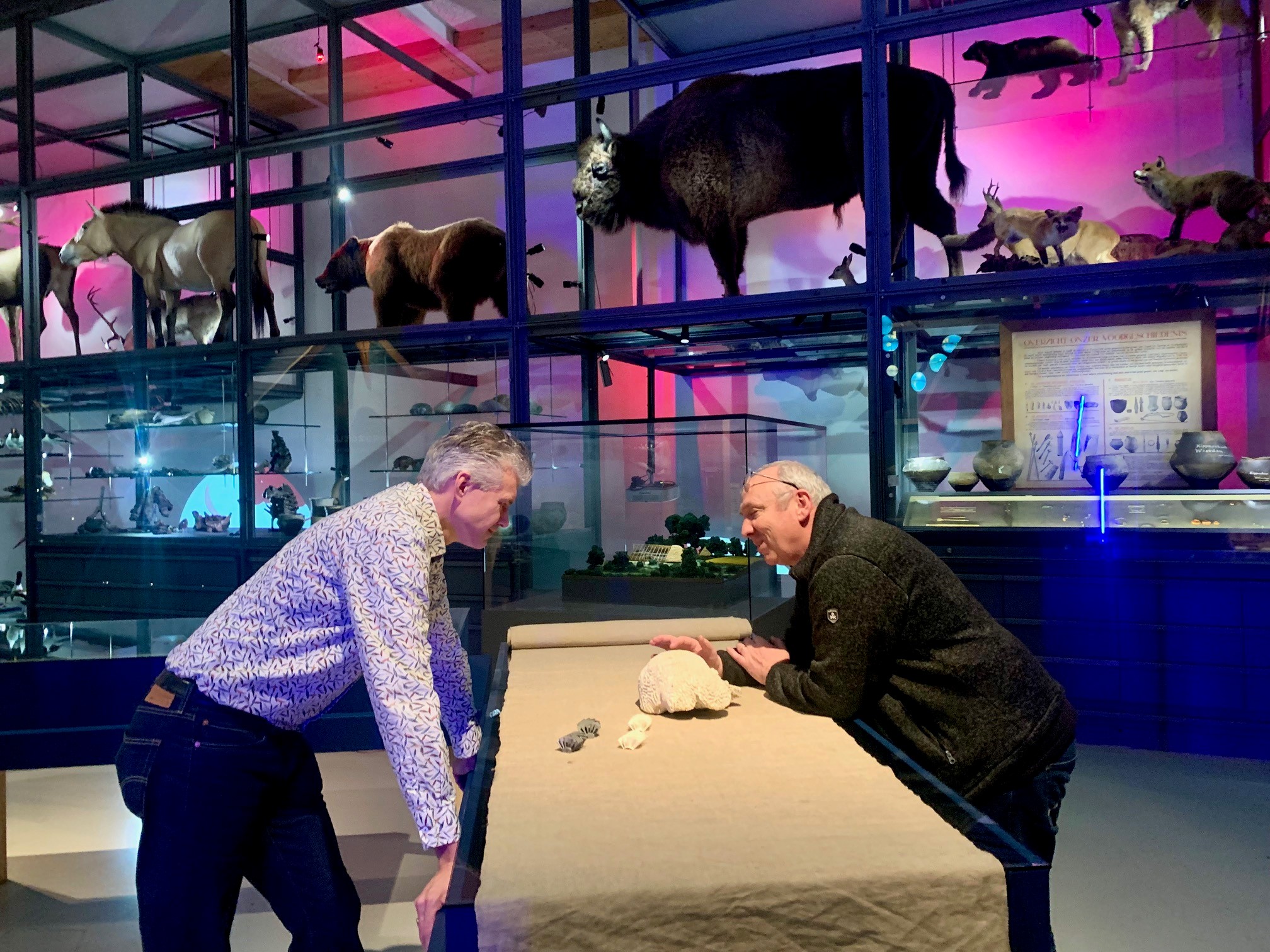
The hidden treasures of the Museumfabriek
Wits is hunched over a table that displays not only his 3D prints, but actual brain coral as well. This calcium skeleton is part of the well-preserved collection of the Museumfabriek (“Museum factory”), a natural history museum in Enschede in the Netherlands.
Curator Edwin Plokker is comparing the organic example with its plastic and aluminium counterparts. Plokker was kind enough to dig through the many cabinets full of hidden treasures in the museum’s temperature-maintained storage, to find more examples of smart thermal regulation in nature to discuss with Wits.
The beautiful, snow-white, taxidermy arctic fox he gently places on the table is one of his favourite examples. “The African fennec fox has massive ears with hardly any fur, larger than its own head, that help it lose heat in a warm environment. This artic fox, at home in freezing temperatures and layers of snow, has tiny ears covered in a very dense coat of fur. As they don’t stick out much from the rest of its head, they don’t cause it to lose any precious heat when hunting for prey in winter. It’s such a smart design.”
Deep-sea fibre optics
His next specimen looks like some sort of fairy’s hat, perplexing all onlookers – but turns out to be a glass sponge. This particular type of deep-sea sponge builds its own skeleton in such a way that it entraps a small crustacean inside for life, living together in symbiosis. That skeleton is made of something that resembles manmade fibre-optic cables – the same cables we use for high-speed internet, for instance – but with better durability. Whereas a lot of heat is needed to make manmade fibre-optic material, these sponges are able to create their organic variety in the dark, cold deep. “These sponges could help us design better fibre-optical materials and networks, and more efficient means of creating them!”
Learning from boundary conditions
It is not just animals, of course. Plant life performs some next level thermal regulation of its own. Plokker presents a dried wood anemone, an early-spring flowering plant native to Europe and western Asia, and points to the heart of the flower. “This anemone blooms much earlier than most flowers, before most insect life is active. You’d think that makes it harder for the flower to get pollinated, but it’s found a solution. It’s an expert at following the sun with the centre of its flower, its white petals reflecting the light right into the yellow core. This quickly becomes one of the warmest spots to be in the entire forest, drawing insect life like you wouldn’t believe. Not just bees, but all kinds of insects will use these flowers to warm up and start their day, accidentally pollinating as they go.”
Nature has limitless designs to offer.
Nodding, Wits points at the leaves of a different dried plant specimen in a cabinet nearby. “In nature, every boundary condition will lead to a differently adapted end result. This might mean having to solve the limitations of early spring for the anemone, or an exceptionally shady spot for a certain tree. All trees of a certain species may have the same basic DNA make-up, but the boundary conditions in which they grow will cause them to look different, their branches to grow in different directions, their leaves to grow different channels, and so on. No brain coral is the same – they adapt to the spot in the ocean where they live. It is of crucial importance that we learn to spot these boundary conditions and understand how they impact natural design, in order to learn from it as engineers. Nature has limitless designs to offer.”